Easy and Simple Undergrad Ochem Synthesis Projects
Abstract
By embedding flow technology in the early phases of academic education, students are exposed to both the theoretical and practical aspects of this modern and widely-used technology. Herein, two laboratory flow experiments are described which have been carried out by first year undergraduate students at Eindhoven University of Technology. The experiments are designed to be relatively risk-free and they exploit widely available equipment and cheap capillary flow reactors. The experiments allow students to develop a hands-on understanding of continuous processing and gives them insights in both organic chemistry and chemical engineering. Furthermore, they learn about the benefits of microreactors, continuous processing, multistep reaction sequences and multiphase chemistry. Undoubtedly, such skills are highly valued in both academia and the chemical industry.
Introduction
Continuous-flow chemistry has rapidly established itself as a go-to technology to carry out difficult-to-handle reagents and reaction conditions [1, 2]. Notable examples where flow has made an undeniable impact are photochemistry [3,4,5], electrochemistry [6,7,8], multiphase reactions [9,10,11,12] and handling of toxic, explosive or other hazardous reagents and intermediates [13,14,15, 16, 17]. Its value has been recognized by the industry as well and many companies are establishing small expert groups specialized in flow chemistry [18, 19]. Hence, there is a high demand for skilled personnel with at least a notion of flow chemistry. It is therefore obvious that flow chemistry courses should be implemented in the academic training of future generations of chemists and chemical engineers [20,21,22,23,24,25,26,27,28,29].
However, flow chemistry courses in the chemistry curricula are actually conspicuous by their absence. Historically, undergraduate teaching laboratories have focused on the use of round-bottom flasks to carry out organic chemistry experiments. Although, it is important for undergraduates to learn to work with this conventional and widely-used labware, it is all too often the only reactor type that students see in their entire education. Herein, we demonstrate that a broader education with regard to reactor technologies should not require a complete rethinking of the classical curriculum nor should it be expensive.
Implementation of flow chemistry in both theoretical and practical courses should be a focus of every chemistry major. Indeed, some reactions are better carried out in a flow reactor. As an example, some of the typical synthetic organic chemistry experiments require long reaction times (several hours or days) which is not always advantageous in a busy teaching schedule. Such reactions can often be accelerated in flow reactors via a strategy called process intensification [30, 31]. This would allow students to carry out more experiments within the given time slot. Moreover, hazardous reactions can be carried out in flow without risk, e.g. by generating in situ small quantities of a toxic substance which is subsequently reacted away in a follow-up transformation. Such experiments provide students with fundamental insights into reaction safety and the development of safe reaction environments. Furthermore, students can get insight in the importance of fundamental transport phenomena, like mass and heat transport. This is especially important for multiphase reaction conditions (gas-liquid or liquid-liquid biphasic reactions) or for exothermic reactions, two notoriously challenging examples to execute and to scale in both academia and industry [32].
As many chemical engineering and chemistry students will pursue a career in industry, where continuous processes are often encountered, it is paramount that students are familiar with the basic principles of continuous manufacturing and flow chemistry. While organic chemistry often lingers with batch processing, chemical engineering often sticks to residence time distribution experiments or the determination of other reactor parameters. By combining organic synthesis with continuous-flow techniques, students from both chemistry and chemical engineering tracks can learn from each other's disciplines, i.e. chemists acquire skills in continuous processing while engineers pick up the benefits for the synthesis of organic molecules. This will create a mutual understanding, fostering more fruitful collaborations in the future.
Herein, we disclose our experience with two flow chemistry experiments which have been implemented in the opening teaching laboratory course at Eindhoven University of Technology. One experiment involves the synthesis of hazardous diazonium salts which can be subsequently reacted away to yield a diazo dye (Solvent Yellow 7). A second experiment involves an organic-aqueous biphasic oxidation of thiols to disulfides and provides insight in segmented flow. Both experiments have been carried out by >400 students in the past 4 years and have been well received.
Experiment 1: Synthesis of solvent yellow 7 in continuous flow
The synthesis of Solvent Yellow 7 involves two distinct steps, including a diazotization to yield the corresponding diazonium compound and a subsequent diazo coupling reaction with phenol to generate the targeted diazo dye (Fig. 1a). This experiment is an example of multistep flow reaction sequences [33,34,35] and shows convincingly how to handle hazardous intermediates. The explosion-sensitive diazonium salts are generated in the first step and immediately reacted away in an electrophilic substitution reaction [36, 37]. Hence, at any given time, the total inventory of the hazardous intermediate is kept small alleviating the safety risks associated with this transformation. The first reaction takes place under acidic conditions, while the second reaction requires basic conditions. All starting materials were selected because of their low cost and relative low toxicity. The concentration of the different solutions is low (C = 0.01 M) which is advantageous both in terms of reduced chemical consumption and enhanced safety.
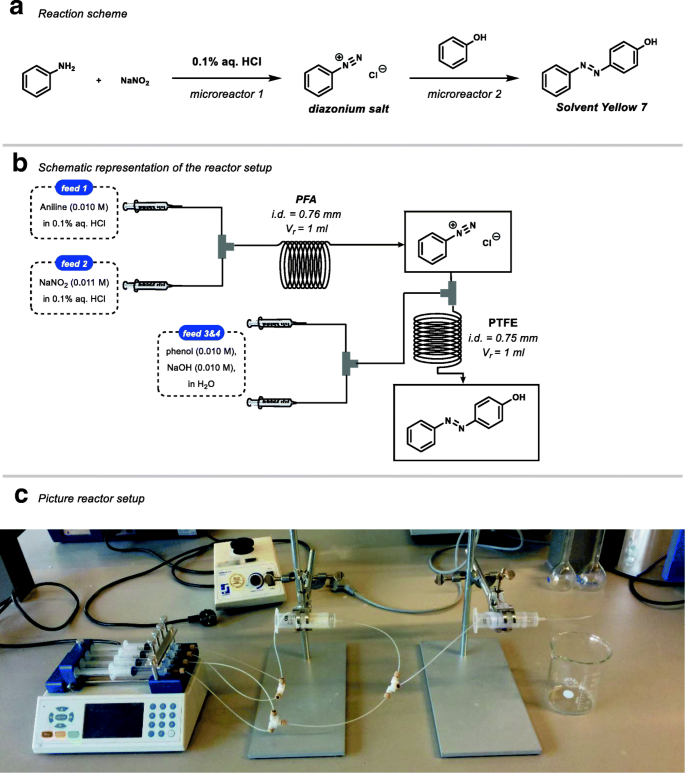
Continuous-flow synthesis of Solvent Yellow 7 via an in-situ generation of diazonium salts. a Reaction scheme. b Flow setup. c Picture of the flow setup
The setup is assembled by the students in pairs as shown schematically in Fig. 1b. The two reactors are made from perfluoroalkoxy alkane (PFA) capillaries (ID = 760 μm, volume 1 mL) according to a procedure described elsewhere (Fig. 1c) [38]. The reactors are connected to the syringe pump, to each other and to the outlet with standard microfluidic fittings. Once the setup is assembled, the different solutions are made with the proper concentrations. To gain time, the solutions can also be made by the supervising tutors. The solutions are taken up in 4 plastic syringes (BD Discardit II®), which are subsequently mounted onto a syringe pump. In addition, the concentrations of the reagents are calculated so that all syringes are pushed at the same flow rate. By doing so, only a single syringe pump is required per experiment, reducing further the cost associated with this experiment. Next, several samples are collected (1 mL/sample) for different residence times (tR = 15, 30, 45, 60, 90, 120, 150 and 300 s). Prior to each sample, students need to wait four residence times in order to ensure steady state data collection. Samples are subsequently diluted and the conversion is measured using a benchtop UV-vis spectrometer (λ = 400 nm).
Next, the students are asked to analyze their results. First, they need to calculate the kinetic constant. The reaction is first order in aniline, which allows us to use the following equation:
$$ -\frac{dC}{dt}= kC $$
(1)
with C, the concentration [M] and t, the reaction time [s]. Integration of this equation leads to:
$$ \ln \left(\frac{1}{1-X}\right)= kt $$
(2)
with
$$ \mathrm{X}=\frac{C_0-{C}_t}{C_0} $$
(3)
The value of the rate constant (k) can be readily obtained by plotting the conversion in function of the residence time (Fig. 2a). Next, ln [1/(1-X)] is plotted in function of the residence time (Fig. 2b). The value for the rate constant can be subsequently derived from its slope, i.e. k = 0.0045 s−1. While slight variations are obtained by different sets of students, the order of magnitude of the rate constant is the same for all.
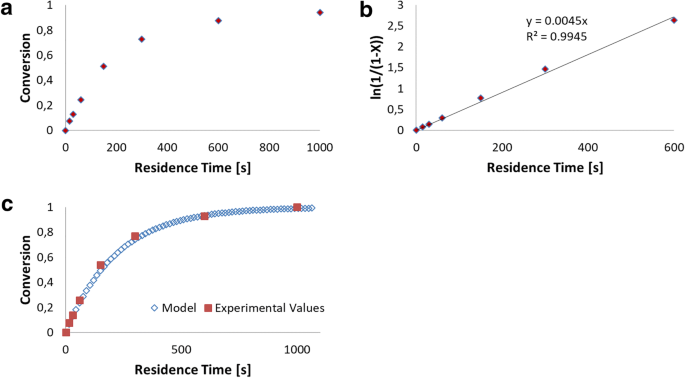
Experimental data for the continuous-flow synthesis of Solvent Yellow 7. a Conversion in function of residence time. The conversion was calculated by dividing the obtained UV-vis absorption (At) at 400 nm by the absorption observed at full conversion (A∞). b Linearization of the obtained data to retrieve the rate constant k. c Plot of equation 4 using the obtained reaction rate k and overlay with the obtained experimental values
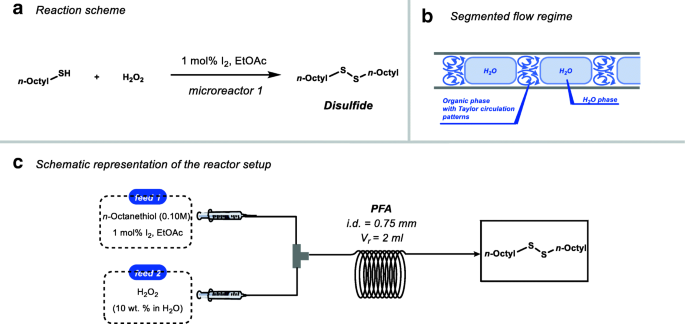
Continuous-flow biphasic synthesis of disulfides using hydrogen peroxide as oxidant. a Reaction scheme. b Schematic representation of the segmented flow regime. c Schematic representation of the flow setup
Next, we ask the students to calculate the time needed to obtain 99% conversion of the starting material. By using equation 2 with X = 0.99, it can be easily understood that tR ≥ 1024 s.
Equation 2 can be reorganized to result into the following equation:
$$ \mathrm{X}=1-{e}^{- kt} $$
(4)
By plotting this equation using the obtained rate constant (Fig. 2c), the conversion can be estimated for all residence times. By adding also the measured datapoints, both theory and experiment can be compared in a single plot (Fig. 2c) and gives confidence to the students that their calculations were accurate.
In principle, the entire experiment requires about four hours of research time per pair of students, this includes setting up the reactor assembly, carrying out the experiments and performing the UV-vis measurements. Since this experiment is done by first year students in the first month of their academic track, we have decided to spread the experiment over two half days. The first day is mainly used to construct the setup and ensuring its proper performance, e.g. no leakage, chemicals mixed in right stoichiometry, calculating the required residence times and getting familiar with the analysis equipment. On the second day the data can be collected and handled. A detailed procedure can be found in the Supporting Information.
Experiment 2: Dimerization of octanethiol in continuous flow
A second experiment was introduced in our undergraduate teaching laboratory to showcase the advantages provided by microreactor technology for the execution of multiphase reaction conditions (Fig. 3). Upon merging two immiscible phases, a segmented flow regime is established in the capillary microreactor where the two phases are brought into close contact with each other [40]. Segmented flow provides fast mixing due so-called Taylor recirculation flow patterns, a well-defined interfacial area and reduced axial dispersion, allowing the reactor to operate as an ideal plug-flow reactor (Fig. 3b).
We selected the synthesis of disulfides via oxidative dimerization of thiols in flow, which has been developed previously in our group using photocatalytic [41,42,43] or electrochemical activation [44]. For the practical course, we wanted a robust, yet operationally simple procedure which avoided the use of mass flow controllers to dose air or oxygen into the liquid stream [45]. A biphasic reaction protocol was developed with 0.1 M octanethiol and 1 mol% I2 in ethyl acetate and a 10% aqueous hydrogen peroxide solution (Fig. 3a). Both solutions are taken up in two syringes and mounted on a single syringe pump (Fig. 3c). Upon merging of the two solutions, a segmented flow regime can readily be observed. The reaction is completed in about 5 min residence time. After discarding the first four reactor volumes to reach steady state, the students are asked to collect sufficient material to isolate the disulfide (20 mL solution, to isolate 1 mmol of the targeted disulfide). This solution is subsequently washed with 0.2 M thiosulfate in a separatory funnel. Note that this is an exothermic reaction which consumes the excess of iodine and thus caution is required. Hence, the combined layers have to be gently shaken and pressure needs to be released from the separatory funnel. The organic phase is subsequently washed two more times with fresh 0.2 M thiosulfate solution. The organic phase is dried with magnesium sulfate and evaporated. The latter step can be preferably done in vacuo using a rotavapor or, since we have >100 students, evaporation can be done in a fume hood by heating the flask in a water bath. The reaction should in principle afford the corresponding disulfide in quantitative yield (276 mg of a transparent oil), but we observed sometimes yields >100% due to insufficient removal of the solvent. All experimental operations can be carried out by the students in four hours.
Assessment
This practical course is for some students their first experimental experience. Consequently, every practical experiment is preceded by an in-depth discussion of the mechanisms of the reactions, the setup, the procedures and the required calculations and plots. This allows teachers to make sure that all students have enough background to carry out the experiments and to understand the observations.
The students are assessed for the experiments in two ways, viz. an evaluation of the practical performance and skills of the student and an objective assessment of the written report. The practical performance is evaluated as follows:
- 1.
Students need to be able to follow the flow procedures accurately, including building up the flow setup, calculating the flow rates correctly to reach certain residence times and executing the recipes with accuracy.
- 2.
They have to abide the safety regulations at all times.
- 3.
They have to be able to accurately note down the made observations and the obtained results in the laboratory notebook.
The written report can be assessed more objectively on its completeness, e.g. accurate description of the observations, correct calculations and plots, adequate conclusion and perspective. We tolerate small mistakes (e.g. rate constant is off), especially when the students assess their performance accurately in the conclusion. Generally, the written reports on the flow experiments are of good to excellent quality. However, it is important to note that the flow experiments are the last ones in the practical course. Hence, the students have by that time already more experience in writing reports and have received ample feedback on previous reports.
Hazards
While microreactors can handle safely small amounts of hazardous chemicals, one should still be careful as the stock solutions and the collecting vessels contain still several mL of solution. Links to appropriate safety hazards data for all chemicals are included in the Supporting Information.
Conclusions
Two laboratory experiments that incorporate microflow techniques were developed for first year undergraduate students at the Eindhoven University of Technology. These experiments are embedded in a general Undergraduate Teaching Laboratory and introduces the concept of continuous-flow processing. It trains the students in a practical manner, which enables them to understand the theory behind the use of flow reactors. The students also get acquainted with building a reactor setup, how to calculate flow rates and convert those to reaction times, reaction stoichiometry and flow synthesis. Importantly, our experiments use cheap and easy-to-operate capillary microreactors and only a single syringe pump per experiment. We believe that these experiments can be readily implemented into both chemistry and chemical engineering curricula.
References
-
Plutschack MB, Pieber B, Gilmore K, Seeberger PH (2017) The Hitchhiker's guide to flow chemistry. Chem Rev 117:11796–11893
-
Govaerts S, Nyuchev A, Noel T (2020) Pushing the boundaries of C–H bond functionalization chemistry using flow technology. J Flow Chem 10:13–71
-
Sambiagio C, Noël T (2020) Flow photochemistry: Shine some light on those tubes! Trends in Chemistry 2:92–106
-
Noël T (2017) A personal perspective on the future of flow photochemistry. J Flow Chem 7:87–93
-
Cambié D, Bottecchia C, Straathof NJW, Hessel V, Noël T (2016) Applications of continuous-flow photochemistry in organic synthesis, material science, and water treatment. Chem Rev 116:10276–10341
-
Noël T, Cao Y, Laudadio G (2019) The fundamentals behind the use of flow reactors in electrochemistry. Acc Chem Res 52:2858–2869
-
Pletcher D, Green RA, Brown RCD (2018) Flow electrolysis cells for the synthetic organic chemistry laboratory. Chem Rev 118:4573–4591
-
Folgueiras-Amador AA, Wirth T (2017) Perspectives in flow electrochemistry. J Flow Chem 7:94–95
-
Liu Y, Chen G, Yue J (2020) Manipulation of gas-liquid-liquid systems in continuous flow microreactors for efficient reaction processes. J Flow Chem 103:103–121
-
Yue J (2018) Multiphase flow processing in microreactors combined with heterogeneous catalysis for efficient and sustainable chemical synthesis. Catal Today 308:3–19
-
Mallia CJ, Baxendale IR (2016) The use of gases in flow synthesis. Org Process Res Dev 20:327–360
-
Ramezani M, Kashfipour MA, Abolhasani M (2020) Minireview: flow chemistry studies of high-pressure gas-liquid reactions with carbon monoxide and hydrogen. J Flow Chem 10:93–101
-
Gutmann B, Cantillo D, Kappe CO (2015) Continuous-flow technology - a tool for the safe manufacturing of active pharmaceutical ingredients. Angew Chem Int Ed 54:6688–6728
-
Movsisyan M, Delbeke EIP, Berton JKET, Battilocchio C, Ley SV, Stevens CV (2016) Taming hazardous chemistry by continuous flow technology. Chem Soc Rev 45:4892–4928
-
Dallinger D, Gutmann B, Kappe CO (2020) The concept of chemical generators: on-site on-demand production of hazardous reagents in continuous-flow. Acc Chem Res 53:1330–1341
-
Gutmann B, Kappe CO (2017) Forbidden chemistries — paths to a sustainable future engaging continuous processing. J Flow Chem 7:65–71
-
Kockmann N, Thenée P, Fleischer-Trebes C, Laudadio G, Noël T (2017) Safety assessment in development and operation of modular continuous-flow processes. React Chem Eng 2:258–280
-
Baumann M, Moody TS, Smyth M, Wharry S (2020) A perspective on continuous flow chemistry in the pharmaceutical industry. Org Process Res Dev. https://doi.org/10.1021/acs.oprd.9b00524
-
May SA (2017) Flow Chemistry, Continuous processing, and continuous manufacturing: a pharmaceutical perspective. J Flow Chem 3–4:137–145
-
Leibfarth FA, Russell MG, Langley DM, Seo H, Kelly LP, Carney DW, Sello JK, Jamison TF (2018) Continuous-flow chemistry in undergraduate education: sustainable conversion of reclaimed vegetable oil into biodiesel. J Chem Educ 95:1371–1375
-
König B, Kreitmeier P, Hilgers P, Wirth T (2013) Flow chemistry in undergraduate organic chemistry education. J Chem Educ 90:934–936
-
Santandrea J, Kairouz V, Collins SK (2018) Continuous flow science in an undergraduate teaching laboratory: Photocatalytic Thiol-Ene reaction using visible light. J Chem Educ 95:1073–1077
-
Kairouz V, Collins SK (2018) Continuous flow science in an undergraduate teaching laboratory: bleach-mediated oxidation in a biphasic system. J Chem Educ 95:1069–1072
-
Simeonov SP, Afonso CAM (2013) Batch and flow synthesis of 5-hydroxymethylfurfural (HMF) from fructose as a bioplatform intermediate: an experiment for the organic or analytical laboratory. J Chem Educ 90:1373–1375
-
Volpe K, Podlesny EE (2020) Modernization of a photochemical reaction for the undergraduate laboratory: continuous flow Photopinacol coupling. J Chem Educ 97:586–591
-
Feng ZV, Edelman KR, Swanson BP (2015) Student-fabricated microfluidic devices as flow reactors for organic and inorganic synthesis. J Chem Educ 92:723–727
-
Vié C, Fattaccioli J, Jacq P (2019) Introduction to droplet-based Millifluidic chemistry using a macroscopic-droplet generator. J Chem Educ 96:797–800
-
Chia MC, Sweeney CM, Odom TW (2011) Chemistry in microfluidic channels. J Chem Educ 88:461–464
-
Hemling M, Crooks JA, Oliver PM, Brenner K, Gilbertson J, Lisensky GC, Weibel DB (2014) Microfluidics for high school chemistry students. J Chem Educ 91:112–115
-
Fernandez Rivas D et al (2020) Process intensification education contributes to sustainable development goals. Part 1. Educ Chem Eng 32 1–14
-
Fernandez Rivas D et al (2020) Process intensification education contributes to sustainable development goals. Part 2. Educ Chem Eng 32 15–24
-
Berton M, de Souza JM, Abdiaj I, McQuade DT, Snead DR (2020) Scaling continuous API synthesis from milligram to kilogram: extending the enabling benefits of micro to the plant. J Flow Chem 10:73–92
-
Britton J, Raston CL (2017) Multi-step continuous-flow synthesis. Chem Soc Rev 46:1250–1271
-
Bloemendal VRLJ, Janssen MACH, van Hest JCM, Rutjes FPJT (2020) Continuous one-flow multi-step synthesis of active pharmaceutical ingredients. React Chem Eng 5:1186–1197
-
Webb D, Jamison TF (2010) Continuous flow multi-step organic synthesis. Chem Sci 1:675–680
-
Oger N, Le Grognec E, Felpin FX (2015) Handling diazonium salts in flow for organic and material chemistry. Org Chem Front 2:590–614
-
Hu T, Baxendale IR, Baumann M (2016) Exploring flow procedures for diazonium formation. Molecules 91:918
-
Straathof NJW, Su Y, Hessel V, Noël T (2016) Accelerated gas-liquid visible light photoredox catalysis with continuous-flow photochemical microreactors. Nat Protoc 11:10–21
-
Britton J, Jamison TF (2017) The assembly and use of continuous-flow systems for chemical synthesis. Nat Protoc 12:2423–2446
-
Noel T, Su Y, Hessel V (2016) Beyond organometallic flow chemistry: the principles behind the use of continuous-flow reactors for synthesis. Top Organomet Chem 57:1–41
-
Talla A, Driessen B, Straathof NJW, Milroy L-G, Brunsveld L, Hessel V, Noel T (2015) Metal-free Photocatalytic aerobic oxidation of Thiols to disulfides in batch and continuous-flow. Adv Synth Catal 357:2180–2186
-
Su Y, Hessel V, Noël T (2015) A compact photomicroreactor design for kinetic studies of gas-liquid photocatalytic transformations. AICHE J 61:2215–2227
-
Bottecchia C, Erdmann N, Tijssen PMA, Milroy L-G, Brunsveld L, Hessel V, Noel T (2016) Batch and flow synthesis of disulfides by visible-light-induced TiO2 Photocatalysis. ChemSusChem 9:1781–1785
-
Laudadio G, Straathof NJW, Lanting MD, Knoops B, Hessel V, Noel T (2017) An environmentally benign and selective electrochemical oxidation of sulfides and thiols in a continuous-flow microreactor. Green Chem 19:4061–4066
-
Su Y, Talla A, Hessel V, Noël T (2015) Controlled Photocatalytic aerobic oxidation of Thiols to disulfides in an energy-efficient Photomicroreactor. Chem Eng Technol 38:1733–1742
Acknowledgments
The execution of a practical course with >100 students per annum is without a doubt a formidable team effort at the department of Chemical Engineering and Chemistry at TU Eindhoven. We would like to thank ir. P. F. A. M. (Peter) Janssens, who is the Director of Education at the department of Chemistry and Chemical Engineering, for his support during the implementation of flow chemistry in the curriculum. Also, we would like to thank all the student assistants in the past five years who helped guiding and supervising this practical course. Finally, we like to thank Ms. Christa Schilders for the administrative tasks during the practicum.
Author information
Authors and Affiliations
Corresponding author
Additional information
Publisher's note
Springer Nature remains neutral with regard to jurisdictional claims in published maps and institutional affiliations.
Electronic supplementary material
Rights and permissions
Open Access This article is licensed under a Creative Commons Attribution 4.0 International License, which permits use, sharing, adaptation, distribution and reproduction in any medium or format, as long as you give appropriate credit to the original author(s) and the source, provide a link to the Creative Commons licence, and indicate if changes were made. The images or other third party material in this article are included in the article's Creative Commons licence, unless indicated otherwise in a credit line to the material. If material is not included in the article's Creative Commons licence and your intended use is not permitted by statutory regulation or exceeds the permitted use, you will need to obtain permission directly from the copyright holder. To view a copy of this licence, visit http://creativecommons.org/licenses/by/4.0/.
Reprints and Permissions
About this article
Cite this article
Kuijpers, K.P.L., Weggemans, W.M.A., Verwijlen, C.J.A. et al. Flow chemistry experiments in the undergraduate teaching laboratory: synthesis of diazo dyes and disulfides. J Flow Chem 11, 7–12 (2021). https://doi.org/10.1007/s41981-020-00118-1
-
Received:
-
Accepted:
-
Published:
-
Issue Date:
-
DOI : https://doi.org/10.1007/s41981-020-00118-1
Keywords
- Flow chemistry
- First-year undergraduate
- Organic chemistry
- Chemical engineering
- Hands-on training
Source: https://link.springer.com/article/10.1007/s41981-020-00118-1
0 Response to "Easy and Simple Undergrad Ochem Synthesis Projects"
Post a Comment